Microscopy just took a major leap forward.
Imagine capturing the invisible: the complex internal structures of living cells, without the need for staining, without harming the samples, and with astonishing precision. Now imagine achieving this with ordinary, incoherent light and using a simple, robust system. This is exactly what our new technique, Incoherent Lateral Shearing Digital Holographic Microscopy (LSDHM), accomplishes.
Our work, published in Optica, redefines what is possible in high-resolution, label-free imaging, opening up new horizons for scientific and clinical research.
The Challenge: Seeing Clearly Without Artifacts
Quantitative phase imaging (QPI) is vital for exploring the structure and behavior of biological samples at the submicrometer scale. However, traditional digital holographic microscopy (DHM) methods depend on coherent illumination, which unfortunately introduces serious drawbacks: speckle noise, parasitic interference artifacts, and a reduction in effective resolution. Commonly used laboratory systems are also highly sensitive to mechanical vibrations and air fluctuations, complicating time-lapse studies and real-world applications.
Using incoherent light sources, like LEDs, has long been an attractive alternative. Yet, achieving single-shot, high-resolution, full-field holography with incoherent illumination remained problematic, mainly due to the difficulty of preserving interference across the entire field of view.
Our Breakthrough: Incoherent Common-Path LSDHM
In our research, we developed a fundamentally new approach: a common-path lateral shearing digital holographic microscope operating in the incoherent regime, where the shearing distance exceeds the coherence area of the illumination source. This breakthrough allows for artifact-free, high-resolution phase imaging without the need for complex two-arm interferometers. By employing a clever arrangement of displaced incoherent beams and a diffraction grating-based shearing module, we enable the full-field interference necessary for phase reconstruction.
The method delivers consistent, high-accuracy quantitative phase reconstructions with excellent stability. We experimentally validated its performance across a wide range of condenser-objective configurations, achieving compatibility with different lateral resolutions and fields of view. Our system was capable of imaging single cells, such as human cheek cells, yeast cells, and diatoms, capturing fine internal structures with unprecedented clarity. Measurements demonstrated remarkable long-term phase stability, with fluctuations below 2 nanometers over 12 minutes even without any active vibration isolation. Phase reconstruction accuracy was also verified against model systems like glass beads, showing excellent agreement with theoretical predictions.
How It Works
The core idea is simple but powerful. Two spatially displaced beams derived from the same incoherent light source pass through the sample and are later interfered by matching the displacement to the shear introduced by a diffraction grating. Unlike classical systems, where temporal and spatial coherence is mandatory for forming an interference pattern, our method relies on carefully controlling the beam geometry rather than the light’s coherence properties. This ensures full-field interference even with low-coherence sources, effectively eliminating traditional speckle and parasitic noise problems. Thanks to the common-path design, the system is inherently resistant to vibrations and environmental fluctuations, enabling stable time-lapse measurements critical for dynamic biological studies.
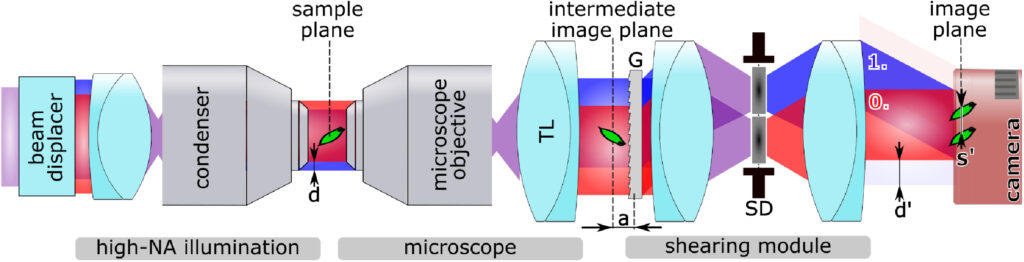
Why It Matters
This work marks a fundamental shift. By freeing high-accuracy phase imaging from the need for highly coherent light, we simplify the hardware, increase robustness, and make real-time label-free imaging much more practical. The method is particularly suited for biomedical applications, where fragile, living samples need to be observed without introducing labeling agents that might disturb natural processes. It also offers significant potential for materials science, microfluidics, and clinical diagnostics, where real-time monitoring of transparent structures is crucial.
Moreover, because the technique is easily scalable and compatible with a wide range of microscope setups, it paves the way for both high-end research instruments and compact, portable devices.
Final Thought: A New Era for Label-Free Imaging
With incoherent lateral shearing digital holographic microscopy, we break the old limitations of traditional lateral-shearing based phase imaging. High-precision, real-time, artifact-free microscopy becomes accessible, practical, and ready for broader applications. This technology opens the door to new discoveries in biology, medicine, and material sciences.
We are excited to share this new possibility with the community and look forward to seeing the many ways it will be applied.
Read our full article in Optica: https://doi.org/10.1364/OPTICA.555786